Research
Installation work for field monitoring on a cut slope of Hokkaido Expressway, September 2016.
Introduction
I worked in many different kinds of geotechnical projects, from anti-seismic retrofitting, ground improvement to permafrost engineering, in different institutions such as Imperial College and Port and Airport Research Institute. At Hokkaido University, my focus is placed more on fundamental characterisation of soil behaviour observed through laboratory experiments, and conceptual/mathematical modelling of the observed behaviour. Meanwhile, more directly field-oriented projects are also constantly undertaken, mainly concernig earth structures such as embankment and levees. To explain the field behaviour, numerical analysis code is also developed by ourselves. This page introduces some of the main research topics from recent years.
Soil Strength and Deformation Characteristics
Quantifying and understand soil's mechanical characteristics, reflected on its strength and deformation, is arguably one of the most fundamental issues in soil mechanics and geotechnical engineering. While the reseach of this traditional topic is becoming mature, it remains extremely difficult to obtain high-quality data on soil's peculiar features through advanced laboratory testing. Sometimes I note that practicing engineers struggled to understand standard trixial testing properly (let alone performing it themselves). Even though the procedures to obtain strength (the angle of the shear resistance and cohesion) and stiffness (E50) have been in every standard book, soil testing is probably inherently complex and delicate. Wrong or inappropriate application of the conditions and outputs is frequently observed in practice. While trying to contribute to maintaining soil testing standard in the geotechnical community, our group seeks to understand soil's important features such as heterogeneity, anisotropy, viscosity, etc. through advanced, sometimes original, laboratory tests. We also work on developing new testing methods that benefit from new inventions of our time.
Heteregeneity, Anisotropy, Viscosity, etc.
Anisotropy of soil has been an evasive issue in geotechnical engineering - everyone knows it, but no one (or few) tries to face it straight (remember St. Augustine's words - When you don't ask, I know it, but when you ask, I don't). In fact, formulae and models to take account of anisotropy have been around for many decades, but somehow they find it difficult to get into daily practice of geotechnical engineering. It has been known, for example, clays tend to be stronger twice and stiffer twice or even three times in the horizontal directions than in the vertical direction (to put in plain terms, that is). Many geotechnical discussions appear to regard the effect 'secondary' - however, discussing a few percents of prediction errors when ignoring anisotropy obviously makes no sense. For soil mechanics to be a precise material science, techniques are required that precisely measure the features that characterise the real soils. This is easier said than done - our group's missions include keeping the highest testing standard, while seeking innovation that allows advanced testing with less specialised equipment.
(left) Compression of peat under isotropic pressure, (right) Internal fabric of peat, 75mm-diameter sample
Peculiarity of soil's deformation characteristics is well recognised when we test peats, for example (though they may be 'atypical' to textbook writers). See in the above photos how a peat sample deforms under isotropic pressure (*1). Significant compression occurs only in the axial (vertical) directions, while the the diameter decreases only slightly. The V/H strain ratio reaches 5 in some cases. The heteregeneity and anisotropy of the internal fabric are obvious in the above X-ray CT image (courtesy of Dr Satoshi Matsumura, PARI), showing horizontal layering of peat fibres, with sporadic twigs (black specks). The horizontal displacement of the ground due to embankment construction, for example, is a great concern - however, knowing to what extent it propagates requires precise knowledge and modelling of the peat behaviour (*2).
(left) Displacement field in peat specimen under one-dimensional compression: See it become more uniform with compression, (right) One-dimensional compression with stepwise changes in the strain rate
The above figures show the response of peat under 1D compression, with the strain rate varied stepwise every 5% strain (*3). This test was undertaken in a special oedometer in which full field displacement was measured by image analysis. At smaller strains, the displacement field is very non-uniform, while it gets more and more uniform as the compression proceeds. With compression, the stress-strain curve becomes more isotach too, suggesting intertwined effects of (non-)uniformity and viscosity. To put in plain words, maybe we can say peats, which are initially non-uniform with many macropores and inclusions, become more 'continuum-like' as they get compressed.
Special triaxial apparatus to obtain anisotropic stiffness parameters
Anisotropy can be significant in more 'conventional' soils - clays, for example. This is the conclusion from my PhD work (anisotropy of London clay), after all. Shown above is special triaxial apparatus at HU for obtaining anisotropic stiffness parameters (*4). It is relatvely straightforward to obtain 'undrained' shear stiffness, but 'drained' (i.e. soil skeletal) stiffness is much harder to observe in clays, which require extremely slow loading, and hence much higher sensor stabilities.
The charts below summarise what we measured so far (*5-7), compared at isotropic stress state (for symbol defintions, see the references). With higher sand content, or with lower concentration of soil particles (as in air foam-mixed cement-treated clay), soil becames more isotropic.
Anisotropy of various soils: Soil is isotropic when all the ratios are 1
*1 Nishimura, S. (2021) New and alternative methods for laboratory characterisation of soils. Bulletin of Japanese Geotechnical Society, Vol.69, No.1, pp.8-12. (in Japanese)
*2 Yamazoe, T., Tanaka, H., Hayashi, H. and Nishimura, S. (2014) A constitutive model formulation for predicting far-field deformation in peaty soft ground and its application to field embankment test. Japanese Geotechnical Journal, 9 (3) 427-442. (in Japanese) DOI: 10.3208/jgs.9.427
*3 Thenuwara, T. A. U, Nishimura, S., Ichikawa, R. (2018) Investigation of the strain rate-dependent consolidation behavior of four peats. Technical Report of JGS Hokkaido Branch, Vol.58, pp.233-242.
*4 Nishimura, S. (2014) Assessment of anisotropic elastic parameters of saturated clay measured in triaxial apparatus: Appraisal of techniques and derivation procedures. Soils and Foundations 54 (3) 364-376. DOI: 10.1016/j.sandf.2014.04.006
*5 Nishimura, S. (2014) Cross-anisotropic deformation characteristics of natural sedimentary clays. Géotechnique 64 (12) 981-996. DOI: 10.1680/geot.14.P.088
*6 Martínez, D. A. and Nishimura, S. (2015) Stiffness anisotropy characteristics of natural fined-grained seabed sediments. 6th International Symposium on Deformation Characteristics of Geomaterials, Buenos Aires, 824-831.
*7 Nishimura, S. and Martínez, D. A. (2016) Cataloguing stiffness anisotropy of natural sedimentary soils – from clays to intermediated soils. Proceedings of the International Mini-Symposium Chubu, Nagoya, Japanese Geotechnical Society Special Publication 5 (2) 101-106. DOI: 10.3208/jgssp.v05.021
Development of new testing techniques
The above data are not readily available in standatd soil laboratories (I'd say, only a handful of laboratories in the world can do the same). We strive to make such experiments more viable in less specialised laboratories. For example, we are exploring a posibility of exchanging the bender elements with disc-shaped piezometric oscillators, which are non-intrusive into a soil specimen and easier to handle. Supported by Dr. Reiko Kuwano and Dr. Masahide Otsubo of the University of Tokyo, we are trying to validate its use to various geomaterials. Application of the disc elements to clays has still been limited (clay has greater damping, and the elastic waves tend to become less clear as they propagate).
Disc-type oscillator in triaxial apparatus: Exchangeable with bender elements.
We are also keen on adopting image-based measurement in soil testing. Our trial with stereophotogrammetry using mid-market compact digital cameras (just like the ones you own privately) succeeded in resolving the displacements in X-Y-Z directions in order of 0.001mm, while correcting for refraction effects of the cell water and cell wall (*8). You need only limited investment in the system (say, $1,000 for two cameras - less than a single LVDT without amplifier!), and a Python script will do the rest. This method is versatile and applicable to different tests, such as triaxial tests and HCA tests. In addition to strain measurement, our work includes developing new loading protocols that allow determination of anisotropic stiffness parameters with less demand for hardware (*9).
(left) Stereophotogrammetry (reconstruction of 3D coordinates by two cameras),
(right) Reference (black points) and reconstructed (yellow points) target cordinates
Example: Radial displacement of a cylindrical polyurethane specimen under unconfined compression: See the effect of end lubrication
If you think stereophotogrammetry entails some complexity, using just a single camera can still be useful in observing what could not be observed in conventional soil tests. The example of non-uniform displacement field seen in a peat specimen mentioned earlier was from an oedometer test with a transparent confining ring, through which images were taken. The same method was also used for cement-treated soils, and confirmed to be effective in avoiding the bedding error in determining 1D compressibility of stiff soils (*10).
Oedometer with transparent ring: The strain can be resolved to order of 0.001% by subpixel PIV
*8 Nishimura, S. (2021) Small-strain measurement in triaxial test by stereophotogrammetry: Principle and Accuracy. Technical Report of JGS Hokkaido Branch, Vol.61. (in Japanese)
*9 Nishimura, S. and Magalona, F. (2020) An alternative method in triaxial tests to obtain cross-anisotropic elastic parameters. Géotechnique Letters 10 (3) 468-477. DOI: 10.1680/jgele.20.00031
*10 Nishimura, S., Iwaki, A., Takashino, S. and Tanaka, H. (2016) Image-based measurement of one-dimensional compressibility in cement-treated soils. Géotechnique 66 (10) 840-853. DOI: 10.1680/jgeot.15.P.218
Frozen Soil Mechanics / Thermal Effects
Frozen soils
Frozen soil mechanics research is one of the main pillars of our group's activity. The fact that Hokkaido is a cold region is just a coincidence - our main focus is on artificial ground freezing as underground construction technique. Frozen soil mechanics has a long history. Well known papers go back to the 1960s or before. However, we believe that a different, updated kind of frozen soil mechanics is required today, when coupled numerical analysis is becoming, or has become, a norm. For example, traditionally frozen soil behaviour is described only by total stress, but this poses a problem in solving a frozen/unfrozen moving boundary problem within a unified analytical framework. Approaches to this advanced problem has been propose (*1-2), but experimental corroboration is still under way. We are working on experiments and modelling, in collaboration with industry and overseas researchers.
Freezing triaxial apparatus, capable of controlling refrigerant circulation and injection rates simultaneously
Miniature freezing pressure cell, in which bender element tests can be performed under isotropic pressure
We developed special freezing triaxial apparatus to investigate the strength and deformation characteristics of frozen soils (*3). This apparatus allows freezing a soil specimen quickly under confining pressure. A few different freezing methods are available, among whch our new invention include quick one-dimensional freezing that yields a uniform triaxial specimen (*4). By adopting a Megatorque Motor, a variety of loading modes can be achieved, including creep tests. With proximity transducers that work in ethelene glycol, accurate measurement of small-strain stiffness is also possible (*5). We also developed a simple miniature pressure cell, in which small-strain stiffness is obtained by bender element tests under isotropic pressure (*6)
1D freeze/thaw device for X-ray CT
Example of mesoscopic soil structure changes during freezing and thawing: When freezing is slow and the confining pressure is low, ice and soil tend to segregate, but otherwise this does not occur.
In addition to the soil behaviour in frozen states, research is also undertaken on the process of freezing and thawing (*7). While frost heave tests have been commonly performed in the context of cold region engineering, these tests are basically model tests (i.e. boundary value problems), not element tests representing the soil behaviour per se. Modelling soil behaviour based on the frost heave test results is therefore a kind of inverse problem, and led to many different, but somewhat similar, models. To advance the research further, we will need to pay more attention to each detail of the soil behaviour during freezing and thawing, rather than simply pursuing to reproduce only the 'overall' results such as frost heave rate (which can be achieved by many different models, in fact). Our recent research includes volume changes during freezing and thawing as soil element, by testing a very small soil specimen that allows relatively uniform temperature and volume change distribution inside. Aided with X-ray CT imaging, we are building an element model that can be incorporated into continuum-based numerical analysis codes. Our eventual goal is to develop a thermo-hydro-mechanical code that can simulate the processes of multi-dimensional ground freezing, excavation and thawing by a single model. A new FEM code has been developed by Python (my own FEM code used to be in C language - COVID-19 ironically gave me time to newly develop it in Python) .
*1 Nishimura, S., Gens, A., Olivella, S. and Jardine, R. J. (2009) THM-coupled finite element analysis of frozen soil: formulation and application. Géotechnique 59 (3) 159-171. DOI: 10.1680/geot.2009.59.3.159
*2 Nishimura, S. and Wang, J. (2019) A simple framework for describing strength of saturated frozen soils as multi-phase coupled system. Géotechnique 69 (8) 659-671. DOI: 10.1680/jgeot.17.p.104
*3 Wang, J., Nishimura, S. and Tokoro, T. (2017) Laboratory study and interpretation of mechanical behavior of frozen clay through state concept. Soils and Foundations 57 (2) 194-210. DOI: 10.1016/j.sandf.2017.03.003
*4 Nagai, Y. and Nishimura, S. (2020) The effect of freezing method on frozen clay strength in triaxial compression test. Technical Report of JGS Hokkaido Branch, Vol.60, pp.1-10.
*5 Wang, J., Nishimura, S., Joshi, B. R. and Okajima, S. (2019) Small-strain deformation characteristics of frozen clay from static testing. Géotechnique. 69 (9) 816-827. DOI: 10.1680/jgeot.18.p.115
*6 Nishimura, S., Wang, J., Okajima, S. and Joshi, B. R. (2019) Small-strain deformation behaviour of a clay at frozen and unfrozen states: A comparative study. 7th International Symposium on Deformation Characteristics of Geomaterials, Glasgow. E3S Web of Conferences 92 04001 – 04001. DOI: 10.1051/e3sconf/20199204001
*7 Nishimura, S., Okajima, S., Joshi, B. R., Higo, Y. and Tokoro, T. (2020) Volumetric behaviour of clays under freeze-thaw cycles. Géotechnique, Ahead of print. DOI: 10.1680/jgeot.20.P.047
Deformation by heating
Thermo-mechanical responses of soil are investigated by looking at heating too, which generally causes volume reduction in soil. Following a potential project of heat-pipe installation in Hokkaido, a preliminary study of heating in peat ground has been initiated. It has been known that heating causes notable contraction in clays, but we noted that the effect is particularly significant in peats. Moderate heating from a room temperature leads to remarkable compression in peats. For example, the figure below shows that nearly 10% strain is observed in step 2 of test 1, where the stress was increased from 10kPa to 30kPa under 10oC. The subsequent step (step 3) raised the temperature from 10oC to 30oC while keeping the stress, and a strain as large as 15% was observed. We are aiming to understand the mechanisms behind this significant effect, as well as modelling the phonomenon for engineering purposes. An eventual goal will be to integrate the 'heating model' with 'freezing/thawing model' to complete the integrated thermo-mechanical model.
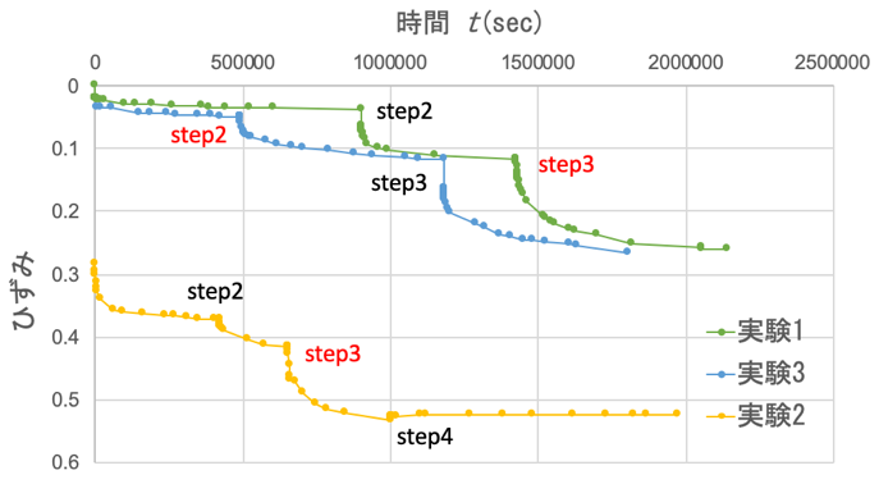
(left) 1D compression of peat: The steps in black letters represent stress increases, while the steps in red letters represent temperature increases, (right) Temperature-controlled oedometer
Field Monitoring of Earth Structures - Hydro-Mechanical Behaviour
Earth structure behaviour during winter
Some say, "No water, no failure in earth structures". Understanding the pore water pressure changes in earth structure in short and long terms is crucially important. Intense rainfalls, long-term climatic variations and local topography and stratigraphy have combined influence on the problem. In cold regions, we also need to take account of snow cover and its melting. When we have an unexpectedly warm day in winter, or encounter relatively heavy (if not torrential) rainfall in spring time when snow is already warming up, earth structures are subject to sudden wetting. Human activities such as removal/heaping of snow add to the complexity. We are apporaching to the problem in two different ways. One is to follow a conventional cycle of monitoring, modelling, and analysis. For this, our group actively work in field, setting up monitoring stations in embankments and levees. We also have home-grown unsaturated seepage analysis code that has a variety of ground-atmosphere interfaces.
(left) Levee under snow cover, (right) Simulation and record of pore water pressure during winter and spring(*1)
Another approach is to detect earth structures' instabilisation by real-time monitoring by transferring the data by wireless remote communication. 'Early warning systems' have been proposed for long - this idea is getting more and more realistic, as the communication network develops and the terminal hardware becomes cheaper. Our group adopts LPWA (Low Power Wide Area) communication technology, and developed a tensiometer array system that measures pore water pressure at four depths and send the data every 30 minutes to the cloud at a cost of around $200. Japan has around 14,000km of river levee under the central government's management - having this system, say, every 200m does not sound unrealistic any more. I personally call such approach as 'IoG - Internet of Ground'. We are preparing for this new era's arrival, and accumulating data and experience (*4).
(left) LPWA-tensiometer array installed at river levee (right) Installation to expressway embankment
Monitoring is easier said than done. If you try youself, you will encounter many unexpected problems. Once I drove a 48.6mm-diameter steel pipe into the slope (as deep as 1m), but I found it completely bent by snow's downward load as it slid down the slope in spring. One of the controllers, which worked perfectly in lab, did not go into a sleep mode and the battery was gone just after one set of data was recorded ..., etc. There are many 'in hindsight ..."s. We are working in field undaunted by severe weather and conditions (once I slid down the slope and smashed my patella).
(left) Sometimes we need to go on snow. Actually, it helps when we have heavy luggage as we can use a sleigh. (right) A fox - looks cuddly, but mind the lethal parasites they carry
Our group's strength lies in ability (or willingness) to make the device ourselves from bits and pieces - or, chips and screws. In addition to the pore water pressure, whatever the physical quantities - ground and snow temperatures, snow cover depth, inclination, etc. -, we add sensors and measure them.
Components in stock for making monitoring devices
River levee stability during heavy rainfall
Apart from the cold region problems, we have a nationwide problem of heavy rainfalls. River levees are eventually bleached by flood waters, either by erosion or seepage, but wetting by rainfall prior to the arrival of flood water is a key factor in initiating the process. Sometimes levees shows a sign of instability even before the river water rise. It is important to predict the response of pore water pressure against heavy rainfall - how quickly and how high the phreatic surface within the river levee will be, and how quicky it recedes. This process is obviously influenced by the climate and ground conditions. For this reason, we have set up monitoring stations at sites under different geographical conditions, such as temperate Akita (*5), cold Hokkaido (*2-3) and tropic Bangkok (*6-7). We are waiting for opportunities to capture unsual heavy rainfalls. So far we observed a response against, for example, 90mm/day. Our air-water coupled numerical analysis indicates that the air phase start affecting the process by being lock up within the levee when the rainfall is greater than, say, 100mm/day. We are maintaining the monitoring station, waiting for the moment(*5).
Cross section of Babame River levee and simulation of the saturation and air pressure against heavy rainfall by air-water coupled seepage analysis
For simulation, we adopted air-water coupled analysis as well as more conventional unsaturated seepage analysis. In the above simulation results, the impedance of air phase to wetting has been considered, as well as hysteresis of the soil water characteristics curve(*5).
*1 地盤工学会北海道支部気候変動に伴う積雪寒冷地の地盤災害リスクに関する研究委員会 (2017) 委員会報告6.2節(西村執筆担当分).気候変動に伴う積雪寒冷地の地盤災害に関するシンポジウム発表論文集 (in Japanese)
*2 Nishimura, S., Tokoro, S. and Rivas, M. F. (2016) Predicting pore water pressure variations in embankments due to evapotranspiration and precipitation. Technical Report of JGS Hokkaido Branch, Vol.56, pp.339-348.
*3 Nishimura, S., Tokoro, T., Yamada, T., Izumi, N. and Rivas, M. F. (2015) A case study of long- and short-term hydraulic state changes in embankment in Hokkaido. 6th Japan-China Geotechnical Symposium, Sapporo, Japanese Geotechnical Society Special Publication 1 (7) 34-39. DOI: 10.3208/jgssp.JPN-38
*4 Nishimura, S., Kawajiri, S. and Yamazoe, N. (2020) Pore water pressure monitoring in river dykes with multi-channel tensiometers with LPWA data-transfer system.第8回河川堤防技術シンポジウム (in Japanese)
*5 Nishiie, S., Nishimura, S. and Yamazoe, N. (2019) Long- and short-term pore water pressure variations in sandy river dike interpreted with 1- and 2-phase seepage flow analysis. 7th Asia-Pacific Conference on Unsaturated Soils, AP-UNSAT 2019, Nagoya. Japanese Geotechnical Society Special Publication 7 (2) 648-653. DOI: 10.3208/jgssp.v07.099
*6 Jotisankasa, A., Pramusandi, S., Nishimura, S. and Chaiprakaikeow, S. (2019) Field response of an instrumented dyke subjected to rainfall. Geotechnical Engineering Journal of the SEAGS & AGSSEA 50 (1) (Online).
*7 Shrestha, A., Jotisankasa, A., Chaiprakaikeow, S., Pramusandi, S., Soralump, S. and Nishimura, S. (2019) Determining shrinkage cracks based on the small-strain shear modulus – suction relationship. Geoscience 9 (9) 362. DOI:10.3390/geosciences9090362
*8 Shinsei, A., Nishimura, S., Fujisawa, K., Takeshita, Y., Kawai, K., Sako, S., Mori, T., Yamazoe, N., Ohta, M. (2019) Insights from round-robin seepage analysis exercise on river levee wetting due to rainfall infiltration. Journal of Japan Society of Civil Engineers, Ser. C (Geosphere Engineering) 75(4), 398-414. (in Japanese) https://doi.org/10.2208/jscejge.75.398
Other Topics - Mixed Soils, New Embankment Soils, etc. -
In addition to the above (or related to the above), research on various different topics is being undertaken. The topics include mechanical and chemical characterisation of steel slag-clay mixture through collaboration with geochemists (*1-3) and of cement-treated, compacted soils with different histories of stress, temperature and moisture (*4-5). The movies below show the difference in response to direct shearing due to different brittleness in steel slag-clay mixture at different ages (X-ray CT images by courtesy of Dr Satoshi Matsumura, PARI).
Direct shear tests on steel slag-clay mixture: (left) 0-day curing, (right) 14-day curing
*1 Toda, K., Kikuchi, R., Otake, T., Nishimura, S., Akashi, Y., Aimoto, M., Kokado, T. and Sato, T. (2020) Effect of soil organic matters in dredged soils to utilization of their mixtures made with a steel slag. Materials 13 (23) 5450. DOI: 10.3390/ma13235450.
*2 Weerakoon, N., Nishimura, S., Sato, H., Toda, K., Sato, T. and Arai, Y. (2020) Stiffness and strength mobilization in steel slag-mixed dredged clays in early curing. Ground Improvement 173 (2) 65-81. DOI: 10.1680/jgrim.17.00083
*3 Toda, K., Sato, H., Weerakoon, N., Otake, T., Nishimura, S. and Sato, T. (2018) Key factors affecting strength development of steel slag-dredged soil mixtures. Minerals 8 (5) 174, DOI: 10.3390/min8050174
*4 Por, S., Nishimura, S. and Likitlersuang, S. (2017) Deformation characteristics and stress responses of cement-treated expansive clay under confined one-dimensional swelling. Applied Clay Science 146 316–324. DOI: 10.1016/j.clay.2017.06.022
*5 Nishimura, S. and Abe, K. (2015) Effects of early-age consolidation on strength development in cement-treated clay. 15th Asian Regional Conference on Soil Mechanics and Geotechnical Engineering, Fukuoka, 2053-2058.