- HOME
- Wow! Cool Laboratory [Researcher Introduction]
- Shingo Matsumoto
Wow! Cool Laboratory [Researcher Introduction]
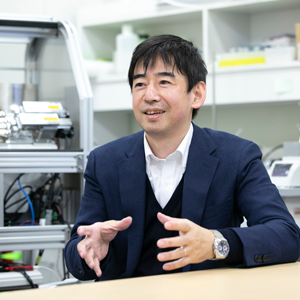
Fields of research: metabolism, quantum chemistry, chemical biology, biomedical engineering, magnetic resonance imaging
Research theme: Development and biomedical application of hyperpolarized NMR/MRI techniques
E-mail: smatsumoto[a]ist.hokudai.ac.jp
Aiming to solve the opposing issues of extending healthy life expectancy and reducing medical expenses
Development of a novel diagnostic MRI technique that relies on metabolic differences associated with diseases
Establishing a “hyperpolarization tag” that eliminates the risk of ionizing radiation exposure
Currently in Japan, which is a ‘super-aging society’, the extension of healthy life expectancy is being advocated, but the increase in national medical expenses related to the elderly is an urgent issue to be solved.
Associate Professor Shingo Matsumoto of the Magnetic Resonance Engineering Laboratory thinks that “early diagnosis and treatment are the keys to solving both issues at the same time”, and he is tackling the task of developing a novel MRI-based molecular imaging technique that can non-invasively visualize shifts in tissue metabolism.
When patients cannot be diagnosed well only by anatomical images that show the morphology of organs, we frequently use nuclear medicine, including PET scans, in which a tracer labeled with a radioisotope is administered to the patient and its distribution is observed.
Prof. Matsumoto says, “Radioisotope labeling is chosen for molecular imaging because the signals obtained from ordinary stable isotopes are too weak to detect. Nuclear medicines using radioisotopes, which afford tens of thousands of times higher sensitivity than stable isotopes, have been considered ideal for molecular imaging. Although the amount of radioisotope is very small, there is a risk of exposure to ionizing radiation for both patients and medical staff, so the frequency of scans for each patient is limited. Therefore, a technology called ‘hyperpolarized MRI’, which enhances the sensitivity of stable isotopes, has been gaining increased attention.”
Each nuclear spin, such as that of a proton (1H) or of carbon 13 (13C), has a property like that of a small magnet, and in a strong magnetic field, its orientation aligns along the north–south pole of the external magnetic field. It can be either of parallel or antiparallel to the N–S direction. “Aligning the directions of the nuclear spins, which are normally disorderly, to either pole in a quantum manner results in substantial enhancement of the NMR/MRI signal, making it feasible to conduct molecular imaging by MRI,” says Associate Professor Matsumoto. “This hyperpolarized stable isotope label is also called a ‘hyperpolarization tag’ (Fig. 1), and clinical trials of hyperpolarized 13C metabolic MRI are under way in more than 10 countries.”
Fig. 1
When hyperpolarized 13C-labeled pyruvate is injected into the body, it is metabolized to water and carbon dioxide in normal tissues, but in cancerous tissues, the pathway is blocked and pyruvate is mainly metabolized to lactate. Cancerous tissues can be identified by the distribution of the corresponding hyperpolarized 13C lactate. In addition, since pyruvate is a glucose metabolite existing endogenously in the body, the risks of side effects from MRI with hyperpolarized 13C pyruvate are quite small, making safer tests feasible.
Developing a room-temperature, low-magnetic-field hyperpolarizer system that anyone can use anywhere
Although dynamic nuclear polarization (DNP) is the most popular technique for inducing hyperpolarization in stable isotopes and is also used for clinical trials, the initial cost of a DNP-based hyperpolarizer device is extremely high—more than a few hundred million yen—because it requires the installation of superconducting magnets of 3T or stronger magnetic field and it requires millimeter-wave irradiation under cryogenic temperatures around 1K in a dedicated space. This high-end nature of DNP-based hyperpolarizers is a barrier to their widespread use.
Associate Professor Matsumoto, who had studied DNP at the US National Cancer Institute for many years, started to develop a low-cost, more versatile hyperpolarizer after arriving at Hokkaido University. He formed a joint research team with Japan Redox Co., Ltd., which develops and manufactures hyperpolarized 1H-MRI for small animals, and with Professor Norihiko Takeda of the Center for Molecular Medicine, Jichi Medical University.
In February 2021, the research team announced the development of a 13C hyperpolarizer system based on the phenomenon known as parahydrogen-induced polarization (PHIP) (Fig. 2), whereby hyperpolarized 13C molecules can be produced at room temperature and under a low magnetic field— that is, in an ordinary room.
“The preparation of hyperpolarized 13C tracers by PHIP goes through two steps. The para-hydrogenation of a multiple bond generates two hyperpolarized 1Hs, and then their quantum state is transferred to the target carbon-13. We’re optimizing the process to make the polarization transfer step more selective and efficient, and we’ve applied for patents on the quantum technology to achieve it.”
Fig. 2
Cell-death imaging achieved using [1-13C]fumarate with 100,000 times the sensitivity
Using the PHIP hyperpolarizer system, Associate Professor Matsumoto and his research team prepared hyperpolarized 13C fumarate, which provides a 13C MRI signal 100,000 times stronger than that at thermal equilibrium. When fumarate is externally administered to a living body, the fumarate is metabolized by the enzyme fumarase, which is released into the extracellular space, but only where there is necrotic cell death with cell membrane disruption. Using the fumarate activity outside the cells, MRI scans of the metabolism of hyperpolarized 13C fumarate administered to a hepatitis mouse model successfully visualized the location of hepatic cell death in the liver for the first time with PHIP (Fig. 3).
The collaborative research is progressing further, with the National Cerebral and Cardiovascular Center and Toray Engineering Co., Ltd. participating as new members. The former of these new members will start research that applies the prototype PHIP hyperpolarizer system to the investigation of cardiac diseases, and the latter will move from the device for preclinical studies to the development of a clinical hyperpolarizer system for humans.
“We still have a long way to go before the system, which prepares MRI tracers, can finally be used for diagnosis in clinics, but I’m confident that we’re steadily moving forward step by step in the right direction. The Division of Bioengineering and Bioinformatics, to which the Magnetic Resonance Engineering Laboratory belongs, has an ideal environment for R&D on medical devices because of human resources who are interested in both engineering and biology. It’s very encouraging that the Hokkaido University Hospital Clinical Research and Medical Innovation Center is located on campus and will serve as a contact point for complicated procedures toward clinical approval.”
Fig. 3
The visualization of necrotic cell death occurring at a particular region where hyperpolarized 13C fumarate administered to a hepatitis mouse model has been metabolized to malate (red).
“In the future, we’d like to bring a hyperpolarized 13C metabolic MRI technique that uses hydrogen gas to the clinical arena and realize safe, cost-effective molecular imaging that avoids exposure to ionizing radiation and that will take the place of nuclear medicine examinations,” says Associate Professor Matsumoto.